The future of the world’s satellite infrastructure depends on a device as old as time: the humble clock.
A mainstay on larger, legacy satellites such as the Global Positioning System (GPS), high-precision atomic clocks have enabled essential navigation and telecommunication functions while providing a timekeeping standard for financial markets, power grids and the internet.
Fundamentally, all clocks measure the passage of time by counting oscillations of a resonator. Grandfather clocks count the oscillations of a pendulum (i.e., the number of times the pendulum arm passes through the vertical), while most modern timepieces count the vibrations of electrified quartz.
But differences in manufacturing, as well as the effects of heat and pressure, can cause resonators likes quartz and pendulums to vary in their oscillation period over time.
Because satellites need to send and measure signals traveling at lightspeed, even the smallest variations in frequency can cause substantial errors; a deviation of one one-thousandth of a second could throw off a GPS route – or a missile’s target – by 300 km.
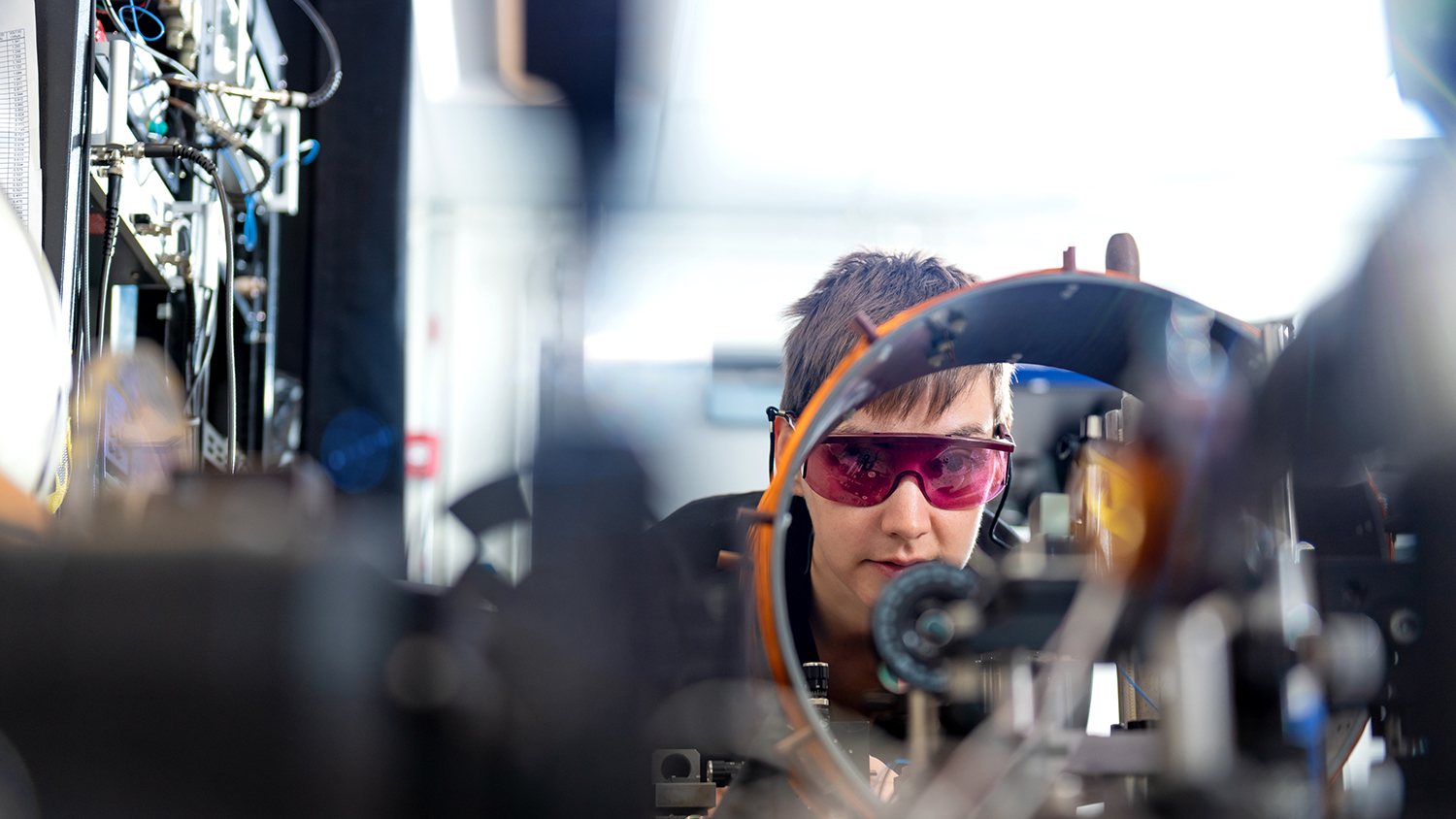
Precision timekeeping could enable swarms of small satellites to communicate with each other more effectively and help spacecraft navigate in deep space. NASA's Jet Propulsion Laboratory is currently testing their Deep Space Atomic Clock, which they hope will assist spacecraft to reach destinations as far as Mars and beyond.
For applications that require this level of precision, clocks aboard satellites must not only be synchronized, but also syntonized, or kept at the same frequency, said James Camparo, an Aerospace Fellow and atomic clock physicist.
Consistency is Key
To maintain syntonization, most atomic clocks employed in space use the same quartz found in other timepieces, but tie the quartz’ oscillation to a fundamental atomic constant of nature, which in principle will not change: the energy-level spacing on an atom’s energy-ladder diagram. Camparo describes the resonant frequency associated with this energy-level spacing as the backbone of ultra-precise timekeeping.
In one type of atomic clock, cesium atoms are subjected to microwave radiation at exactly 9,192,631,770 Hz – a frequency generated by a quartz oscillator. If the quartz oscillator produces this exact frequency, the cesium atoms will change from one rung on their energy-ladder to another.
After the initial cesium-atom/microwave interaction, a detector in the clock measures how many atoms changed rungs. If most of the atoms have jumped from one rung of the energy-ladder to another, the frequency of the microwaves – and by extension, the quartz oscillator – is correct.
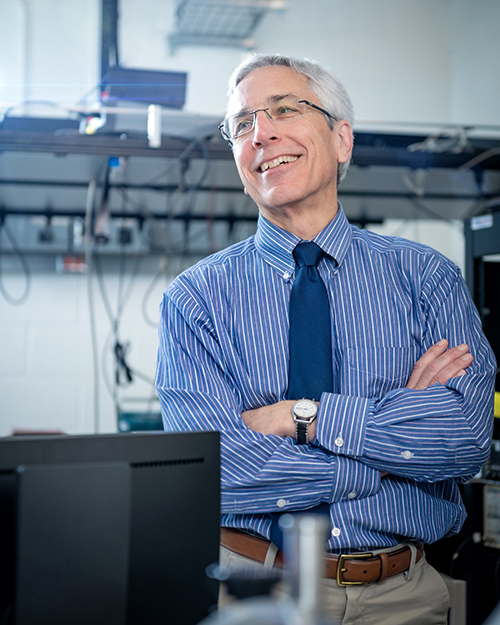
If the atoms have not jumped a rung on their energy-ladder, the frequency has drifted – factors such as temperature or pressure may have altered the quartz, just as they do in everyday timepieces. When this happens, an electric circuit in the clock will correct the oscillator frequency back to 9,192,631,770 Hz.
While most atomic clocks follow this scheme, some use different elements, such as rubidium, and different forms of radiation. The result is the same: precision timekeeping that can self-correct.
Bridging the Divide
As the scientific community works toward smaller, higher precision devices, Camparo and his team conduct critical research on alternative clock designs and study the long-term impact of environmental factors on timekeeping through simulation and testing.
Working in parallel with a commercial manufacturer developing a new laser-based clock, the team is currently evaluating the efficacy of a simplified version with a lower-powered laser – a design that could significantly extend the life of the device.
“If we succeed, we can pass that information through the open literature to the manufacturer. If we fail, we haven’t interrupted the contractor’s progress and in fact, we can alert the timekeeping community not to go down that path,” Camparo said. “This is how Aerospace’s research – success or failure – can be of benefit to America’s place in space.”
Ultimately, the lab’s strength in foundational research, combined with Aerospace’s public interest mission, enable it to bridge the divide within the atomic clock community.
“We take on problems that academia might not be interested in and manufacturers don’t have the time and resources to look at,” he said. “If you don’t know the basic atomic physics, you may find an engineering solution, but it may not be the optimal solution.”